Matthew VanBrocklin
Associate Professor of Surgery and Adjunct Associate Professor of Oncological Sciences
Melanoma, Carcinoma, Non-Small-Cell Lung, Molecular Targets
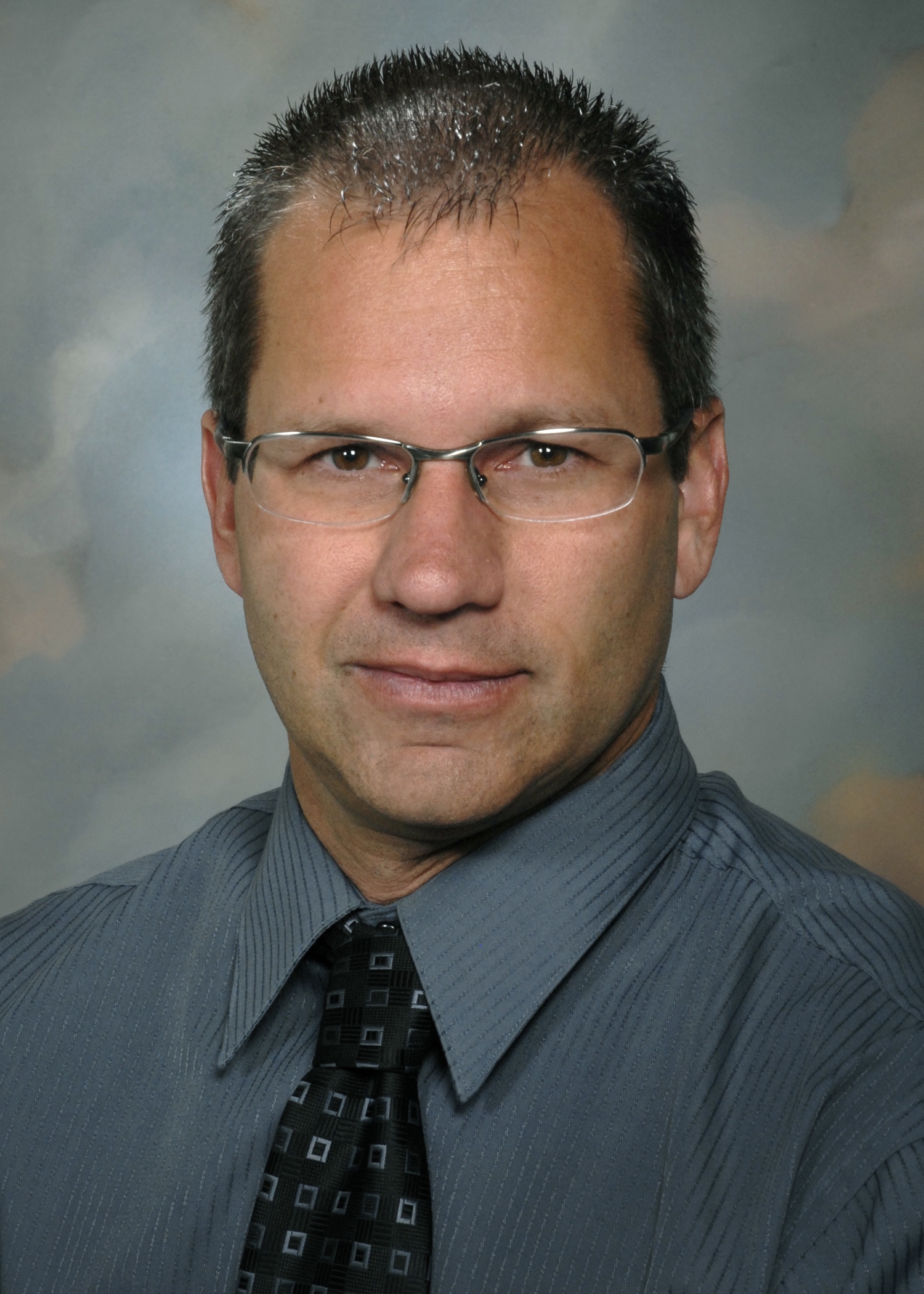
Molecular Biology Program
Education
B.S. Western Michigan University
M.S. Mayo Graduate School
Ph.D. Western Michigan University
Research
My research program has four major areas of focus: (1) Utilizing our RCAS/TVA model in order to identify novel drivers of melanoma (e.g., NF-1, KIT, RAC1, PDGFRA), identify resistance mechanisms, and to develop more effective therapeutic strategies. (2) Developing CRISPR/Homology Directed Repair (HDR) for in vivo cancer and disease modeling. (3) Characterizing immune checkpoint ligands and evaluating their role in immune evasion in melanoma with long term goals of developing therapeutic agents. (4) Oncolytic Immunotherapy, focusing on developing/evaluating novel oncolytic viruses in combination with immune checkpoint blockade in vivo.
1) RCAS-TVA Model
We are currently evaluating a role for RAC1P29S in melanoma initiation and progression in BRAF or NRAS driven melanomas. Preliminary data suggests that RAC1 is insufficient as a melanoma driver in the context of Cdkn2a and/or Pten loss, but may enhance metastatic progression of BRAF driven tumors. We are assessing a role for RAC1 in promoting metastatic dissemination in our NRAS and Nf-1 melanomas as these alterations co-occur in patients (13-14%). Utilizing in vivo CRISPR targeting Nf-1 (exon 2 or 4) we were successful in inducing tumors in our mouse model in the context of Cdkn2aand/or Pten loss with high penetrance. We are expanding this model to assess pharmacologic and immunotherapeutic intervention strategies.
2) CRISPR/HDR Modeling
We have developed a novel method for single vector CRISPR mediated homology directed repair (HDR) that has high potential in developing novel disease models and gene therapy applications. As proof of principle, we have evaluated the ability of our CRISPR/Cas9-HDR vector to correct altered GFP (possessing an early termination codon) in vitro. We observed a 23% repair rate with our single vector design comparable to current state of the art approaches requiring multiple factors including a ssDNA oligo (17.7%). We have also observed similar results with another CRISPR nuclease hAsCpf1 (15%). We are in the process of applying our technology to developing preclinical models of cancer (e.g., NSCLC, melanoma) and correcting monogenic diseases (e.g., CTFR).
3) Evaluating B7-H3 and B7-H4
We have observed that each of these immune checkpoint ligands is vital for immune evasion in a murine syngeneic tumor model where loss of either (via CRISPR) results in a 0% (B7-H3, 0/51 - B7-H4 0/37) take rate compared to parental melanoma cells with a 60% take rate in these immune competent mice. We are characterizing their role in immune evasion and utilizing novel methods to identify their receptors on CD8+ T cells. We have also begun to develop antibodies that block the interaction of these immune checkpoint ligands with their receptors in order to block immunosuppressive signaling.
4) Oncolytic Immunotherapy
We have developed several novel oncolytic viruses and are evaluating them in several preclinical cancer models. These are wild type RNA viruses that utilize cellular receptors often overexpressed on many tumor types. Therefore, their tropism is largely limited to specific tumor cells enhancing viral infection and spread while limiting toxicity. We are evaluating these as single agents and in combination with immune checkpoint blockade. We are generating modified viruses with expanded tropism by altering capsid genes. We are also developing replication competent viruses encoding small immunomodulatory factors that may enhance host antitumor immunity. In addition, in collaboration with Dr Robert Andtbacka we are evaluating longitudinal patient samples treated with oncolytic viruses (HF10, CVA21) in order to identify biomarkers of response and/or resistance.
References
- Aspirin suppresses PGE2 and activates AMP kinase to inhibit melanoma cell motility, pigmentation and selective tumor growth in vivo. Grossman D, Kumar D, Rahman H, Tyagi E, Liu T, Li C, Lu R, Lum D, Holmen SL, Maschek JA, Cox JE, and VanBrocklin MW DOI: 10.1158/1940-6207.CAPR-18-008, 2018 Jul 18.
-
A phase I trial of azacitidine and nanoparticle albumin bound paclitaxel in patients with advanced or metastatic solid tumors. Cohen AL, Ray A, VanBrocklin MW, Burnett DM, Bowen RC, Dyess DL, Butler TW, Dumlao T, Khong HT. Oncotarget. 2016 Dec 26;8(32):52413-52419. DOI: 10.18632/oncotarget.14183. eCollection 2017 Aug 8. PMID: 28881739
-
Resistance mechanisms to genetic suppression of mutant NRAS in melanoma. Robinson JP, Rebecca VW, Kircher DA, Silvis MR, Smalley I, Gibney GT, Lastwika KJ, Chen G, Davies MA, Grossman D, Smalley KSM, Holmen SL, VanBrocklin MW. Melanoma research. 2017; 27(6):545-557. NIHMSID: NIHMS905926 PubMed [journal] PMID: 29076949 PMCID: PMC5683096
-
A phase I study of intratumoral ipilimumab and interleukin-2 in patients with advanced melanoma. Ray A, Williams MA, Meek SM, Bowen RC, Grossmann KF, Andtbacka RH, Bowles TL, Hyngstrom JR, Leachman SA, Grossman D, Bowen GM, Holmen SL, VanBrocklin MW, Suneja G, Khong HT. Oncotarget. 2016; 7(39):64390-64399. PubMed [journal] PMID: 27391442 PMCID: PMC5325451
-
AKT1 Activation Promotes Development of Melanoma Metastases. Cho JH, Robinson JP, Arave RA, Burnett WJ, Kircher DA, Chen G, Davies MA, Grossmann AH, VanBrocklin MW, McMahon M, Holmen SL. Cell reports. 2015; 13(5):898-905. NIHMSID: NIHMS726140 PubMed [journal] PMID: 26565903 PMCID: PMC4646731
-
Model of the TVA Receptor Determinants Required for Efficient Infection by Subgroup A Avian Sarcoma and Leukosis Viruses. Melder DC, Pike GM, VanBrocklin MW, Federspiel MJ J Virol. 2015 Feb 15;89(4):2136-48.
-
Akt signaling accelerates tumor recurrence following ras inhibition in the context of ink4a/arf loss. Robinson GL, Robinson JP, Lastwika KJ, Holmen SL, Vanbrocklin MW. Genes Cancer. 2013 Nov;4(11-12):476-85.
-
Ink4a/Arf loss promotes tumor recurrence following Ras inhibition. Vanbrocklin MW, Robinson JP, Lastwika KJ, McKinney AJ, Gach HM, Holmen SL. Neuro Oncol. 2012 Jan;14(1):34-42.
-
Activated MEK cooperates with Ink4a/Arf loss or Akt activation to induce gliomas in vivo. Robinson JP, Vanbrocklin MW, Lastwika KJ, McKinney AJ, Brandner S, Holmen SL. Oncogene. 2011 Mar 17;30(11):1341-50.
-
Akt signaling is required for glioblastoma maintenance in vivo. Robinson JP, Vanbrocklin MW, McKinney AJ, Gach HM, Holmen SL. Am J Cancer Res. 2011;1(2):155-167.
-
Phase II trial to evaluate gemcitabine and etoposide for locally advanced or metastatic pancreatic cancer. Melnik MK, Webb CP, Richardson PJ, Luttenton CR, Campbell AD, Monroe TJ, O'Rourke TJ, Yost KJ, Szczepanek CM, Bassett MR, Truszkowski KJ, Stein P, Van Brocklin MW, Davis AT, Bedolla G, Vande Woude GF, Koo HM. Mol Cancer Ther. 2010 Aug;9(8):2423-9.
-
Phase II trial to evaluate gemcitabine and etoposide for locally advanced or metastatic pancreatic cancer. Melnik MK, Webb CP, Richardson PJ, Luttenton CR, Campbell AD, Monroe TJ, O'Rourke TJ, Yost KJ, Szczepanek CM, Bassett MR, Truszkowski KJ, Stein P, VanBrocklin MW, Davis AT, Bedolla G, Vande Woude GF, Koo HM. Mol Cancer Ther. 2010 Aug;9(8):2423-9. doi: 10.1158/1535-7163.MCT-09-0854. Epub 2010 Aug 3. PMID: 20682649
-
Targeted delivery of NRASQ61R and Cre-recombinase to post-natal melanocytes induces melanoma in Ink4a/Arflox/lox mice. VanBrocklin MW, Robinson JP, Lastwika KJ, Khoury JD, Holmen SL. Pigment Cell Melanoma Res. 2010 Aug;23(4):531-41.
-
Activated BRAF induces gliomas in mice when combined with Ink4a/Arf loss or Akt activation. Robinson JP, VanBrocklin MW, Guilbeault AR, Signorelli DL, Brandner S, Holmen SL. Oncogene. 2010 Jan 21;29(3):335-44.
-
Met amplification and tumor progression in Cdkn2a-deficient melanocytes. Vanbrocklin MW, Robinson JP, Whitwam T, Guilbeault AR, Koeman J, Swiatek PJ, Vande Woude GF, Khoury JD, Holmen SL. Pigment Cell Melanoma Res. 2009 Aug;22(4):454-60.
-
Mitogen-activated protein kinase inhibition induces translocation of Bmf to promote apoptosis in melanoma. VanBrocklin MW, Verhaegen M, Soengas MS, Holmen SL. Cancer Res. 2009 Mar 1;69(5):1985-94.
-
Inhibition of avian leukosis virus replication by vector-based RNA interference. Chen M, Granger AJ, Vanbrocklin MW, Payne WS, Hunt H, Zhang H, Dodgson JB, Holmen SL. Virology. 2007 Sep 1;365(2):464-72.
-
Differential oncogenic potential of activated RAS isoforms in melanocytes. Whitwam T, Vanbrocklin MW, Russo ME, Haak PT, Bilgili D, Resau JH, Koo HM, Holmen SL. Oncogene. 2007 Jul 5;26(31):4563-70.
-
A novel BH3 mimetic reveals a mitogen-activated protein kinase-dependent mechanism of melanoma cell death controlled by p53 and reactive oxygen species. Verhaegen M, Bauer JA, Martín de la Vega C, Wang G, Wolter KG, Brenner JC, Nikolovska-Coleska Z, Bengtson A, Nair R, Elder JT, Van Brocklin M, Carey TE, Bradford CR, Wang S, Soengas MS. Cancer Res. 2006 Dec 1;66(23):11348-59.
-
Antibody microarray profiling reveals individual and combined serum proteins associated with pancreatic cancer. Orchekowski R, Hamelinck D, Li L, Gliwa E, VanBrocklin M, Marrero JA, Vande Woude GF, Feng Z, Brand R, Haab BB. Cancer Res. 2005 Dec 1;65(23):11193-202.
-
Simplified method for the construction of gene targeting vectors for conditional gene inactivation in mice. Wang PF, Kong D, Van Brocklin MW, Peng J, Zhang C, Potter SJ, Gao X, Teh BT, Zhang N, Williams BO, Holmen SL. Transgenics. 2005 4(3):215-8.
-
Mitogen-activated protein kinase pathway-dependent tumor-specific survival signaling in melanoma cells through inactivation of the proapoptotic protein bad. Eisenmann KM, VanBrocklin MW, Staffend NA, Kitchen SM, Koo HM. Cancer Res. 2003 Dec 1;63(23):8330-7.
-
Apoptosis and melanogenesis in human melanoma cells induced by anthrax lethal factor inactivation of mitogen-activated protein kinase kinase. Koo HM, VanBrocklin M, McWilliams MJ, Leppla SH, Duesbery NS, Vande Woude GF. Proc Natl Acad Sci USA. 2002 Mar 5;99(5):3052-7.
-
Capsid-targeted viral inactivation can eliminate the production of infectious murine leukemia virus in vitro. VanBrocklin M, Federspiel MJ. Virology. 2000 Feb 1;267(1):111-23.
-
Extended analysis of the in vitro tropism of porcine endogenous retrovirus. Wilson CA, Wong S, VanBrocklin M, Federspiel MJ. J Virol. 2000 Jan;74(1):49-56.
-
The EV-O-derived cell line DF-1 supports the efficient replication of avian leukosis-sarcoma viruses and vectors. Schaefer-Klein J, Givol I, Barsov EV, Whitcomb JM, VanBrocklin M, Foster DN, Federspiel MJ, Hughes SH. Virology. 1998 Sep 1;248(2):305-11.
-
Expression of a murine leukemia virus Gag-Escherichia coli RNase HI fusion polyprotein significantly inhibits virus spread. VanBrocklin M, Ferris AL, Hughes SH, Federspiel MJ. J Virol. 1997 Apr;71(4):3312-8.
-
Efficient lipid-mediated transfection of DNA into primary rat hepatocytes. Holmen SL, Vanbrocklin MW, Eversole RR, Stapleton SR, Ginsberg LC. In Vitro Cell Dev Biol Anim. 1995 May;31(5):347-51.